Fuel cells convert the chemical energy of a fuel, such as hydrogen, into electrical energy very efficiently on many size scales, without combustion and with little or no emission of pollutants. They are therefore suitable in a variety of applications utilizing clean power generation.
Solid Oxide Cells (SOCs) are highly efficient, entirely solid-state fuel cells that operate at high temperatures. SOCs have numerous applications including stationary power, vehicular auxiliary power, and energy storage. SOCs are a highly scalable technology that gives the capability to meet power requirements ranging from large-scale centralized power generation to distributed generation in individual homes and businesses. As depicted in Figures 1 and 2, SOCs can operate in either 1) Fuel cell or 2) Electrolysis modes.
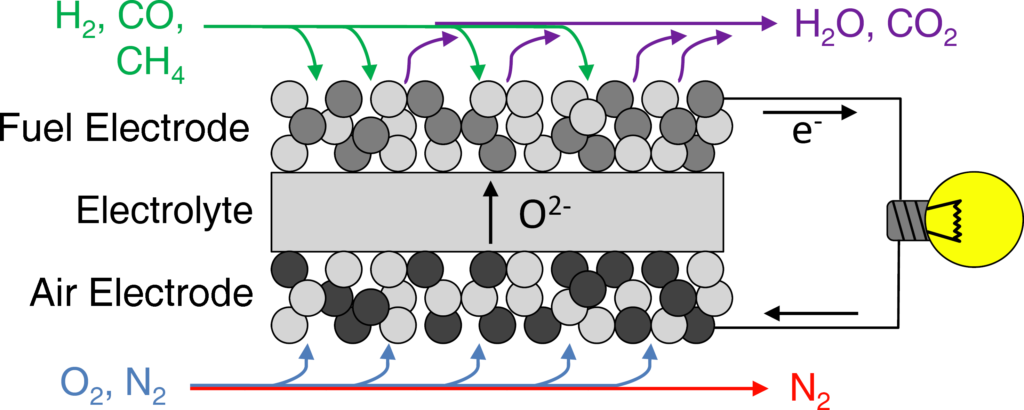
Figure 1: SOC in fuel cell mode. Fuel gases and oxygen are converted to electrical energy and heat with fuel-to-electricity efficiencies of up to 60%.
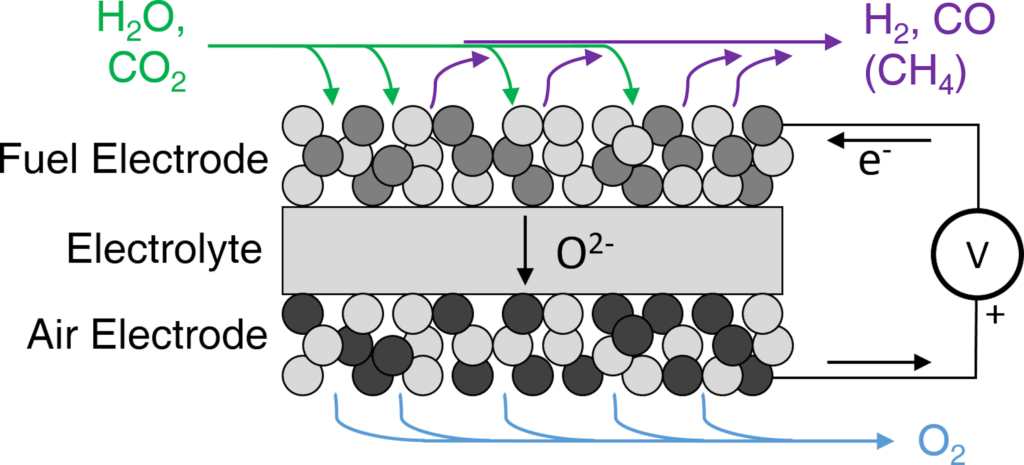
Figure 2: SOC in electrolysis mode. Electrical energy and heat are converted to fuel gases and oxygen with efficiencies up to 100% even at practical production rates.
SOCs are composed of three layers: a fuel electrode, an electrolyte, and an air electrode. Both the fuel and air electrodes are porous and they conduct both ions and electrons. The electrolyte is an ionic conductor and electronic insulator.
On the fuel electrode side during fuel cell mode, the fuel (for example hydrogen) diffuses through the porous anode to the interface between the fuel electrode and the electrolyte – the reaction sites, commonly known as the triple phase boundaries (TPBs). The fuel reacts with the oxygen ions that are conducted through the oxide electrolyte to produce water and electrons. Since the electrolyte is an electronic insulator, the electrons travel through the electronic conductor in the anode, commonly nickel. At the surface of the fuel electrode the electrons are collected by the current collector, resulting in electrical current being transmitted to the external circuit. On the air electrode side, the electrons from the external circuit combine with the oxygen molecules in the air to produce oxygen ions. The oxygen ions are then conducted through the electrolyte back to the fuel electrode side.
SOCs can have several different designs. The most common designs are the planar design and the tubular design. The planar design has a lower manufacturing cost and a higher energy density. In the planar design, the cells (anode-electrolyte-cathode) are stacked together, separated by an interconnect plate. The interconnect is used as a current collector and also as a gas manifold for the fuel and air electrodes.
Benefits of SOCs
- No air pollutants (SOx, NOx, CO, and particulates)
- High electrical efficiency (50-60%) & corresponding low CO2 emissions that are separate from the airstream and therefore easier to sequester than in a combustion process
- High-temperature waste heat useable for co-generation of electricity (with gas or steam turbines) for ~70% system electrical efficiency
- Low noise and no pollution allows urban siting, thereby allowing additional use of waste heat for hot water or space heating, for > 90% overall system efficiencies and reduced transmission losses
- Fuel flexibility – SOCs can utilize a wide range of locally – available fuels compatible with both current and future sustainable energy infrastructure, including energy–dense liquid fuels:
- Ethanol from agricultural waste or fast-growing crops (no energy-intensive water removal required as for ethanol combustion)
- Methane from landfill gas, biogas, or natural gas
- Hydrogen from electrolysis with renewable electricity
- CO, H2S, volatile organics (for pollution clean-up)
- Conversion of high grade heat and clean electricity to chemical energy when operated in electrolysis mode.
Challenges for increasing commercial viability
- Lowering cost
- Increasing durability and reliability
Objectives
Our researchers apply principles of materials, mechanical, and chemical engineering to improve the durability and performance and lower the cost of SOCs, with the overall objective of contributing to environmental sustainability.